Time domain reflectometry for dielectric characterization of olive mill wastewater contaminated soils
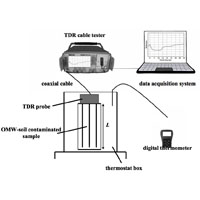
Published:21 December 2020
Abstract Views: 1681
PDF: 496
HTML: 14
HTML: 14
Publisher's note
All claims expressed in this article are solely those of the authors and do not necessarily represent those of their affiliated organizations, or those of the publisher, the editors and the reviewers. Any product that may be evaluated in this article or claim that may be made by its manufacturer is not guaranteed or endorsed by the publisher.
All claims expressed in this article are solely those of the authors and do not necessarily represent those of their affiliated organizations, or those of the publisher, the editors and the reviewers. Any product that may be evaluated in this article or claim that may be made by its manufacturer is not guaranteed or endorsed by the publisher.
Similar Articles
- Vincenzo Bagarello, Vito Ferro, Dennis Flanagan, Predicting plot soil loss by empirical and process-oriented approaches. A review , Journal of Agricultural Engineering: Vol. 49 No. 1 (2018)
- Mario Pirastru, Marcello Niedda, Mirko Castellini, Effects of maquis clearing on the properties of the soil and on the near-surface hydrological processes in a semi-arid Mediterranean environment , Journal of Agricultural Engineering: Vol. 45 No. 4 (2014)
- Costanza Di Stefano, Vito Ferro, Establishing soil loss tolerance: an overview , Journal of Agricultural Engineering: Vol. 47 No. 3 (2016)
- Vidas Damanauskas, Algirdas Janulevičius, Effect of tillage implement (spring tine cultivator, disc harrow), soil texture, forward speed, and tillage depth on fuel consumption and tillage quality , Journal of Agricultural Engineering: Vol. 53 No. 3 (2022)
- Ugo Lazzaro, Caterina Mazzitelli, Benedetto Sica, Paola Di Fiore, Nunzio Romano, Paolo Nasta, On evaluating the hypothesis of shape similarity between soil particle-size distribution and water retention function , Journal of Agricultural Engineering: Vol. 54 No. 4 (2023)
- Abderrahman Sghaier, Hanen Dhaou, Lassaad Jarray , Zouhair Abaab, Ahmed Sekrafi, Mohamed Ouessar , Assessment of drought stress in arid olive groves using HidroMORE model , Journal of Agricultural Engineering: Vol. 53 No. 1 (2022)
- Alessio Cappelli, Lucrezia Lupori, Enrico Cini, Should extra virgin olive oil production change the approach? A systematic review of challenges and opportunities to increase sustainability, productivity, and product quality , Journal of Agricultural Engineering: Vol. 54 No. 1 (2023)
- Lei Liu, Xianliang Wang, Xiaokang Zhong, Xiangcai Zhang, Yuanle Geng, Hua Zhou, Tao Chen, Design and experiment of furrow side pick-up soil blade for wheat strip-till planter using the discrete element method , Journal of Agricultural Engineering: Vol. 55 No. 1 (2024)
- Salahudin Zahedi, Kaka Shahedi, Mahmod Habibnejad Rawshan, Karim Solimani, Kourosh Dadkhah, Soil depth modelling using terrain analysis and satellite imagery: the case study of Qeshlaq mountainous watershed (Kurdistan, Iran) , Journal of Agricultural Engineering: Vol. 48 No. 3 (2017)
- Maria Lisa Clodoveo, Tiziana Dipalmo, Cristina Schiano, Domenico La Notte, Sandra Pati, What’s now, what’s new and what’s next in virgin olive oil elaboration systems? A perspective on current knowledge and future trends , Journal of Agricultural Engineering: Vol. 45 No. 2 (2014)
You may also start an advanced similarity search for this article.